Primary and Metastatic Brain Tumors
Intracranial neoplasms can arise from any of the structures or cell types present in the cranial vault, including the brain, meninges, pituitary gland, skull, and even residual embryonic tissue.
Overview
Intracranial neoplasms can arise from any of the structures or cell types present in the cranial vault, including the brain, meninges, pituitary gland, skull, and even residual embryonic tissue. The overall annual incidence of primary brain tumors in the United States is approximately 20 cases per 100,000 person-years. In 2015, it is estimated that cancer of the brain and central nervous system (CNS) will be diagnosed in 22,850 men and women. The 5-year relative survival rate following this diagnosis is 33.8%.
The most common primary brain tumors are meningiomas, representing 34.7% of all primary intracranial tumors. Gliomas are the second most common primary brain tumor, at 30%, and account for 80% of malignant primary intracranial tumors. In children, primary brain tumors are the most common of the solid tumors and the second most frequent cause of cancer death after leukemia.
Brain metastases (secondary brain tumors) occur in approximately 15% of cancer patients as a result of hematogenous dissemination of systemic cancer, and the incidence may be rising because of better control of systemic disease. Lung and breast cancers are the most common solid tumors that metastasize to the CNS. Proportionate to their incidence, melanoma and testicular and renal carcinomas have the greatest propensity to metastasize to the brain, but their relative rarity explains the low incidence of these neoplasms in large series of patients with brain metastases. Patients with brain metastases from nonpulmonary primaries have a 70% incidence of lung metastases. Although many physicians presume that all brain metastases are multiple, in fact, half are single and many are potentially amenable to focal therapies.
Epidemiology
Gender
There is a slight predominance of malignant primary brain tumors in men, although meningiomas are more likely to develop in women.
Age
Primary brain tumors have a bimodal distribution, with a small peak in the pediatric population and a steady increase in incidence with age, beginning at age 20 years and reaching a maximum of 20 cases per 100,000 person-years between the ages of 75 and 84 years.
Etiology and Risk Factors
The cause of primary brain tumors is unknown, although genetic and environmental factors may contribute to their development.
Genetic Factors
Clear heritable factors play a minor role in the genesis of primary brain tumors; less than 5% of patients with glioma have a family history of brain tumor. Several inherited diseases, such as tuberous sclerosis, neurofibromatosis type I, Turcot syndrome, and Li-Fraumeni cancer syndrome, predispose patients to the development of gliomas. However, these tumors tend to occur in children or young adults and do not account for the majority of gliomas that appear in later life. A large genome-wide association study identified five risk loci for glioma susceptibility: 5p15.33 (TERT), 8q24.21 (CCDC26), 9p21.3 (CDKN2A-CDKN2B), 20q13.33 (RTEL1), and 11q23.3 (PHLDB1).
Molecular Markers
One historical prognostic marker, the epigenetic silencing of the MGMT (O6-methylguanine-DNA methyltransferase) DNA-repair gene by promoter methylation, is an independent prognostic factor in patients with glioblastoma and has been associated with longer survival in patients who received the alkylating agent temozolomide. Epidermal growth factor receptor (EGFR) amplification and overexpression are present in about 60% of glioblastomas. Mutations of the isocitrate dehydrogenase genes (IDH1 and IDH2) predict prolonged progression-free and overall survival, probably as a consequence of identifying a separate class of lesions. Shown to be early glioma-initiating events, IDH gene mutations are present in the majority of World Health Organization (WHO) grade II and III gliomas as well as secondary glioblastoma, but they are rare in primary glioblastoma. Molecular markers of brain tumors can predict survival and will become increasingly important in the diagnosis and treatment of glioma.
Environmental Factors
Prior cranial irradiation is the only well-established environmental risk factor for intracranial neoplasms.
Lifestyle Characteristics
Brain tumors are not associated with lifestyle characteristics such as cigarette smoking, alcohol intake, and cellular phone use.
Signs and Symptoms
Brain tumors produce both nonspecific and specific signs and symptoms.
Nonspecific Symptoms
Nonspecific symptoms include headaches, which occur in about half of patients but are rarely an isolated finding of intracranial tumors, and nausea and vomiting, which are caused by an increase in intracranial pressure. Because of the widespread availability of CT and magnetic resonance imaging (MRI), papilledema is now seen in less than 10% of patients, even when symptoms of raised intracranial pressure are present.
Specific Signs and Symptoms
Specific signs and symptoms are usually referable to the particular intracranial location of the tumor and are similar to the signs and symptoms of other intracranial space-occupying masses.
Lateralizing signs
Lateralizing signs, including hemiparesis, aphasia, and visual-field deficits, are present in approximately 50% of patients with primary and metastatic brain tumors.
Seizures
Seizures are a common presenting symptom, occurring in approximately 25% of patients with high-grade gliomas, at least 50% of patients with low-grade tumors, and 50% of patients with metastases from melanoma, perhaps because of their hemorrhagic nature. Otherwise, seizures are the presenting symptom in 15% to 20% of patients with brain metastases. Seizures may be generalized, partial, or focal.
Stroke-like presentation
Hemorrhage into a tumor may present like a stroke, although the accompanying headache and alteration of consciousness usually suggest an intracranial hemorrhage rather than an infarct. Hemorrhage is usually associated with high-grade gliomas, occurring in 5% to 8% of patients with glioblastoma. However, oligodendrogliomas have a propensity to bleed, and hemorrhage occurs in 7% to 14% of these low-grade neoplasms. Sudden visual loss and fatigue may be seen with bleeding into or infarction of pituitary tumors, termed pituitary apoplexy.
Altered mental status
Approximately 75% of patients with brain metastases and as many as half of patients with malignant gliomas have impairment of consciousness or cognitive function. Some patients with multiple bilateral brain metastases may present with an altered sensorium as the only manifestation of metastatic disease; this finding can be easily confused with metabolic encephalopathy.
Screening for Metastatic Brain Tumors
Screening for brain metastases is performed in only a few clinical situations.
Lung Cancer
Approximately 10% to 15% of patients with small-cell lung cancer have brain metastases at diagnosis, and such metastases develop in more than 45% of patients during their illness. Therefore, cranial CT or MRI is performed as part of the initial evaluation for extent of disease.
Occasionally, patients with non–small-cell lung cancer (NSCLC) undergo routine cranial CT or MRI before definitive thoracotomy, because the presence of brain metastases may influence the choice of thoracic surgical procedure. This approach is particularly valuable in patients with suspected stage IIB or III disease for whom thoracotomy is considered following neoadjuvant therapy.
Diagnosis
Radiographic Appearance of Primary Lesions
MRI
The diagnosis of a brain tumor is best made by cranial MRI. This should be the first test obtained in a patient with signs or symptoms suggestive of an intracranial mass. MRI is superior to CT and should always be obtained with and without contrast material such as gadolinium.
FIGURE 1
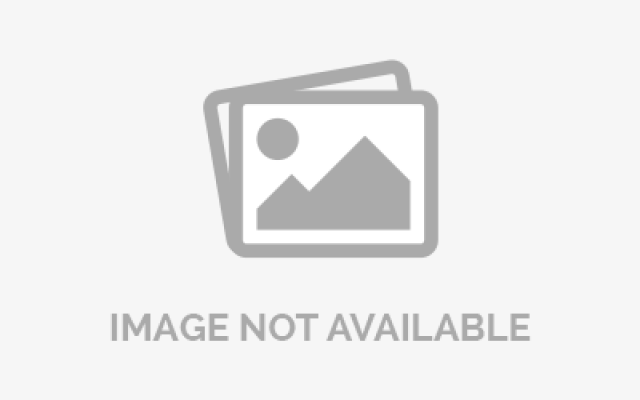
T1-weighted MRI with gadolinium contrast showing a typical appearance of a glioblastoma. Non–contrast-enhanced images of this lesion (not shown) revealed the presence of some hemorrhaging.
High-grade or malignant primary brain tumors appear as contrast-enhancing mass lesions that arise in white matter and are surrounded by edema (Figure 1). Multifocal malignant gliomas are seen in ~5% of patients.
Low-grade gliomas typically are nonenhancing lesions that diffusely infiltrate and tend to involve a large region of the brain. Low-grade gliomas are usually best appreciated on T2-weighted or fluid-attenuated inversion recovery (FLAIR) MRI scans (Figure 2). As many as 40% of nonenhancing tumors may harbor foci of high-grade glioma.
CT
A contrast-enhanced CT scan may be used if MRI is unavailable or if the patient cannot undergo MRI. It is important to note that a pacemaker is not always a contraindication to MRI, and some centers will have pacemaker protocols wherein patients without high-degree heart block may, with the approval of their cardiologist, undergo imaging with resetting of their pacemaker afterward. CT is adequate for excluding brain metastases in most patients, but it can miss low-grade tumors or small lesions located in the posterior fossa. Tumor calcification is often better appreciated on CT than on MRI.
FIGURE 2
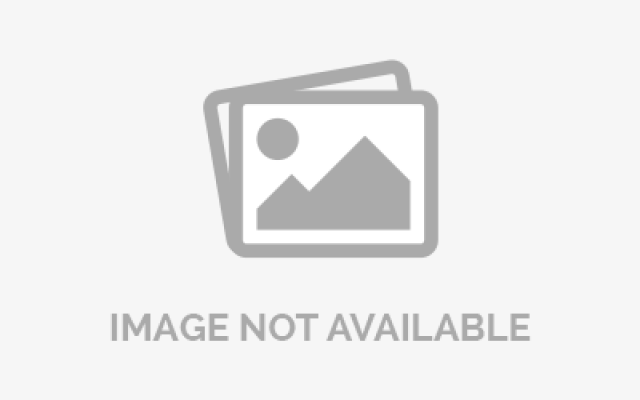
FLAIR MRI demonstrating a diffusely infiltrating, low-grade oligodendroglioma involving the right frontal, insular, and temporal lobes. This lesion did not enhance with gadolinium.
PET
Body positron emission tomography (PET) scans performed for staging of systemic malignancies have a sensitivity of only 75% and a specificity of 83% for identification of cerebral metastases. Therefore, in this setting they are less accurate than MRI, which remains the gold standard.
Radiographic appearance of metastatic lesions
On CT or MRI, most brain metastases are enhancing lesions surrounded by edema, which extends into the white matter (Figure 3). Unlike primary brain tumors, metastatic lesions rarely involve the corpus callosum or cross the midline.
The radiographic appearance of brain metastases is nonspecific and may mimic other processes, such as infection. Therefore, the CT or MRI scan must always be interpreted within the context of the clinical picture of the individual patient, because cancer patients are particularly vulnerable to opportunistic CNS infections or may develop second primaries, which can include primary brain tumors.
Other imaging tools
Magnetic resonance spectroscopy (MRS) and perfusion imaging can help differentiate low-grade from high-grade brain tumors but cannot distinguish different tumor types of the same grade.
Pathology
Glial tumors arise from astrocytes, oligodendrocytes, or their precursors and exist along a spectrum of malignancy. The astrocytic tumors are graded, using the four-tier WHO system. Grade I tumors are localized tumors such as pilocytic astrocytomas or gangliogliomas, which are usually found in children and younger adults. Grade II tumors are low-grade diffuse astrocytomas. Grade III (anaplastic astrocytoma) and IV (glioblastoma) tumors are high-grade malignant neoplasms. Grading is based on pathologic features, such as endothelial proliferation, cellular pleomorphism, mitoses, nuclear atypia, and necrosis. The oligodendroglial neoplasms are classified as either low-grade oligodendroglioma (grade II) or anaplastic oligodendroglioma (grade III).
FIGURE 3
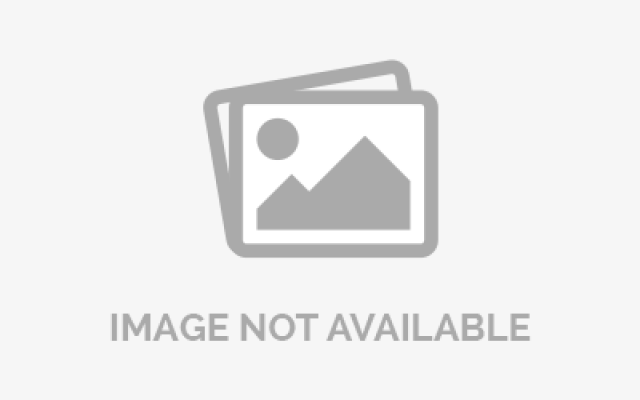
Gadolinium-enhanced MRI scan demonstrating multiple brain metastases. Note the edema surrounding each lesion.
Low-grade glial tumors (such as astrocytoma and oligodendroglioma) and mixed neuronal-glial tumors (such as ganglioglioma) grow slowly but have a propensity to transform into malignant neoplasms over time. Transformation is usually associated with progressive neurologic symptoms and the appearance of enhancement on MRI.
The high-grade gliomas include glioblastoma, gliosarcoma, anaplastic astrocytoma, and anaplastic oligodendroglioma. These tumors are extremely invasive, with tumor cells often found up to 4 cm away, and even on the contralateral side of the brain from the primary tumor.
Ependymoma
Intracranial ependymomas are relatively rare, accounting for less than 2% of all brain tumors. They are most frequently seen in the posterior fossa or spinal cord, although they may also arise in the supratentorial compartment. Ependymomas are typically low grade histologically, but their high rate of recurrence indicates malignant behavior.
Medulloblastoma
Medulloblastomas are uncommon in adults but are one of the two most common primary brain tumors in children (the other being cerebellar astrocytomas). Medulloblastomas arise in the cerebellum and are always high-grade neoplasms.
Two studies developed distinct molecular classifications of medulloblastoma seen in both children and adults. Northcott and colleagues used integrative genomics to identify the following four specific molecular variants of medulloblastoma: WNT, SHH, group C, and group D. These four types had distinct subgroup-specific demographics, histology, metastatic status, and DNA copy number aberrations. SHH tumors were seen in infants and adults, whereas WNT and group D tumors were seen among all ages. Immunohistochemistry for DKK1 (WNT), SFRP1 (SHH), NPR3 (group C), and KCNA1 (group D) could appropriately classify medulloblastomas in approximately 98% of patients. Group C tumors peaked in childhood, were not seen in adults, and conferred the poorest prognosis, independent of metastatic status. Remke and colleagues used gene expression profiling to reveal three distinct molecular variants of adult medulloblastoma, with distinct demographics, genetics, transcriptome, and prognostic implications: SHH, WNT, and subtype D. Both overall survival and progression-free survival were superior for WNT-driven tumors and intermediate for SHH-driven tumors, while subtype D tumors trended toward shorter survival. In a nonrandomized phase II trial, 70 patients 70 years and older with a Karnofsky Performance Status (KPS) score of less than 70 were given temozolomide alone (150 to 200 mg/m2 daily for 5 days every 4 weeks) until progression. Grade 3 to 4 neutropenia and thrombocytopenia occurred in 13% and 14% of patients, respectively. Median progression-free survival time was 16 weeks and median overall survival was 25 weeks; compared with historical controls, these outcomes were significantly greater than those associated with supportive care alone. Eighteen patients (26%) increased their KPS score to 70 or greater.
Primitive Neuroectodermal Tumors
Primitive neuroectodermal tumors (PNETs) are high-grade, aggressive tumors that usually occur in children. They include pineoblastoma and neuroblastoma. Histologically, they are identical to medulloblastomas, but their prognosis is usually worse than that of medulloblastomas. Thus, their biology is different, even though they may be similar pathologically.
FIGURE 4
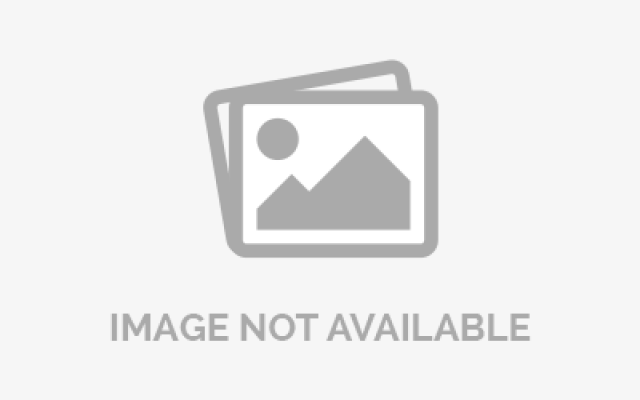
T1-weighted MRI with gadolinium contrast showing the typical appearance of a meningioma. Note the dural-based “tail.”
Extra-Axial Tumors
The most common extra-axial tumor is the meningioma. Meningiomas are usually benign tumors that arise from residual mesenchymal cells in the meninges. They produce neurologic symptoms by compressing the underlying brain. Meningiomas rarely are malignant or invade brain tissue (Figure 4).
Other common extra-axial tumors include pituitary adenoma, epidermoid or dermoid tumors, and acoustic neuroma (vestibular schwannoma). Most extra-axial tumors have a benign histology but can be locally invasive. Many extra-axial benign tumors are incidentally identified on MRI performed for nonspecific symptoms, such as headache. Most of these tumors do not require therapy and do not enlarge. Many benign extra-axial tumors identified in this way can be observed with serial imaging studies alone.
Metastatic Brain Tumors
The pathology of metastatic brain lesions recapitulates the pathology of the underlying primary neoplasm. This feature often enables the pathologist to suggest the primary source in patients whose systemic cancer presents as brain metastasis. However, even after a complete systemic evaluation, the site of the primary tumor remains unknown in 10% to 15% of patients with brain metastases.
Staging and Prognosis
Staging
Staging is not applicable to most primary brain tumors because they are locally invasive and do not spread to regional lymph nodes or distant organs. Staging with an enhanced complete spinal MRI and cerebrospinal fluid (CSF) evaluation is important for a few primary tumor types, such as medulloblastoma, ependymoma, and PNET, because they can disseminate via the CSF. All systemic cancers are stage IV when they present with a brain metastasis.
Prognostic Factors
For most patients with primary brain tumors, prognosis is inversely related to pathologic grade and patient age, and is directly related to the overall clinical condition at diagnosis. Several molecular markers that correlate well with prognosis have been identified, such as mutations of the IDH1 gene in the majority of low-grade gliomas and loss of heterozygosity on chromosomes 1p and 19q, which is characteristic of oligodendroglioma.
Several studies have identified glioblastoma subtypes on the basis of gene expression profiles: proneural, neural, classic, and mesenchymal. Proneural tumors are more likely to carry mutations of IDH1 and p53 and are associated with improved overall survival. Classic tumors frequently (95% of the time) demonstrate EGFR amplification, while mesenchymal tumors are most likely to have mutations or deletions of NF1, often combined with inactivation of PTEN. Classic tumors also have a higher activity of mesenchymal and astrocytic markers CD44 and MERTK, as well as high expression of CHI3L and MET, and are associated with the shortest overall survival time.
The Cancer Genome Atlas (TCGA) presented its most recent update on the landscape of somatic mutations in glioblastoma in 2013. Several recurrently mutated genes were identified, as well as complex rearrangements of genes for signature receptors including EGFR and alpha-type platelet-derived growth factor receptor (PDGFRA). Telomerase reverse transcriptase (TERT) promoter mutations correlated with elevated mRNA expression, supporting a role in telomerase reactivation. Additionally, survival advantage of the proneural subtype was conferred by the glioma CpG island methylator phenotype (G-CIMP). Finally, DNA methylation of the O-6-methylguanine-DNA methyltransferase (MGMT) gene may be a predictive biomarker for chemotherapeutic treatment response.
The importance of the mutation in IDH1 at arginine 132 (IDH1R132MUT) has now been well established. Glioblastomas arising with and without the IDH1 mutation appear to be distinct entities arising from separate cells of origin. It is thought that EGFR amplification or PTEN loss leads to IDH1 wild-type glioblastoma, while a non–stem cell neural precursor in the frontal lobe that undergoes IDH1 mutation and the CpG island methylator phenotype (CIMP) status is the initial lesional event in the development of IDH1-mutant glioma. IDH1 mutant enzyme, when introduced into human astrocytes, induces DNA hypermethylation, resulting in changes seen in CIMP-positive grade II and III gliomas.
IDH mutation is a more powerful prognosticator than WHO grade in grade II and III astrocytomas. IDH-mutant tumors had TP53 mutations, PDGFRA overexpression, PTEN promoter methylation, and prolonged survival, whereas IDH wild-type tumors were associated with EGFR amplification, PTEN loss, and shorter survival.
With conventional treatment, including surgical resection, radiotherapy, and chemotherapy with the alkylating agent temozolomide, median survival time for patients with grade IV glioblastoma ranges from 14 to 18 months. In contrast, low-grade gliomas can be associated with a survival approaching a decade or more; however, patients 40 years or older with low-grade glioma generally have a more aggressive disease and their median survival time is usually less than 5 years. Oligodendroglial histology is favorable, and survival of patients with grade III disease can range from 7 years to more than 14 years if there is 1p/19q codeletion.
For a large proportion of patients with brain metastases, median survival is only 4 to 6 months after whole-brain radiotherapy. However, some patients (ie, those who are younger than 60 years, have a single lesion, or have controlled or controllable systemic disease) can achieve prolonged survival, and these individuals warrant a more aggressive therapeutic approach. Furthermore, most of these patients qualify for vigorous local therapy for their brain metastases, such as surgical resection or stereotactic radiosurgery. These approaches can achieve a median survival of 40 weeks or longer, and rarely some patients are cured.
Treatment
Treatment of primary brain tumors and brain metastases consists of both supportive and definitive therapies.
Supportive Therapy
Supportive treatment focuses on relieving symptoms and improving the patient’s neurologic function. The primary supportive agents are anticonvulsants and corticosteroids.
Anticonvulsants
Anticonvulsants are administered to the 25% of patients who have a seizure at presentation. Traditionally, phenytoin was the most commonly used medication, but carbamazepine and valproic acid are equally effective. Doses of all these anticonvulsants can be titrated to the appropriate serum levels to provide maximal protection.
Anticonvulsants such as levetiracetam, gabapentin, lamotrigine, and topiramate are preferred and have become the first choice for treatment of many patients. Most of these agents have the advantages of causing fewer cognitive adverse effects and less induction or inhibition of the hepatic microsomal system, so they are less likely to alter the metabolism of chemotherapeutic agents. Serum levels of these agents are less reliable than those of older drugs. These agents should replace the older drugs as first-line antiepileptic therapy in most patients.
Prophylaxis. Prospective studies have failed to show the efficacy of prophylactic anticonvulsants for patients with brain tumors who have not had a seizure. Consequently, prophylactic anticonvulsants should not be administered, except during the perioperative period, when their use may reduce the incidence of postoperative seizures; the drugs can be tapered off within 2 weeks of surgery. Increasingly, the new agents are being used for prophylaxis.
Corticosteroids
Corticosteroids reduce peritumoral edema, diminishing mass effect and lowering intracranial pressure. This effect produces prompt relief of headache and improvement of lateralizing signs. Dexamethasone is the corticosteroid of choice because of its minimal mineralocorticoid activity. The starting dose is approximately 16 mg/day, but this dose is adjusted upward or downward to reach the minimum dose necessary to control neurologic symptoms. In patients whose MRI is suggestive of CNS lymphoma, urgent biopsy should precede the initiation of corticosteroid therapy.
Long-term corticosteroid use is associated with hypertension, diabetes mellitus, a nonketotic hyperosmolar state, myopathy, weight gain, insomnia, and osteoporosis. Thus, the corticosteroid dose in patients with a brain tumor should be tapered as rapidly as possible once definitive treatment has begun. Most patients can stop taking corticosteroids by the time they have completed cranial irradiation. All patients who take corticosteroids for more than 6 weeks should receive antibiotic prophylaxis for Pneumocystis jiroveci (formerly carinii) pneumonia. Prophylaxis should continue for 1 month after the corticosteroids have been discontinued. This practice is without supporting evidence (as shown in a large Cochrane review), although it is routinely used.
Definitive Therapy: Primary Brain Tumors
Definitive treatment of brain tumors includes surgery, radiation therapy, and chemotherapy. The first step is to devise an overall therapeutic plan that should outline the sequence and elements of multidisciplinary therapy.
Surgery
Various surgical options are available, and the surgical approach should be carefully chosen to maximize tumor resection while preserving vital brain structures and minimizing the risk of postoperative neurologic deficits. The goals of surgery include (1) obtaining an accurate histologic diagnosis, which in the modern era involves obtaining sufficient tissue for molecular diagnostics; (2) reducing tumor burden and associated mass effect caused by the tumor and/or peritumoral edema; (3) maintaining or re-establishing pathways for CSF flow; (4) conferring a survival benefit by gross total removal; and (5) reducing tumor burden before adjuvant irradiation or chemotherapy. Surgery for a primary brain tumor rarely achieves cure but can reduce tumor burden so that the tumor becomes more amenable to adjuvant irradiation or chemotherapy. In glioblastomas, resection of greater than 98% of enhancing tumor, as measured by postoperative MRI, is associated with improved survival. Similarly, markedly prolonged survival has also been demonstrated for low-grade gliomas and IDH1-mutant gliomas when gross total resection is achieved. Limitations of stereotactic biopsy are that small volumes of tissue are obtained and that tissue sampling errors may result in a failure to reach a correct diagnosis. Stereotactic biopsy may be nondiagnostic in 3% to 8% of cases and has a surgical morbidity of approximately 5%.
Sidebar:Using data from more than 700 newly diagnosed glioblastoma patients, Marko and colleagues developed a mathematical model that revealed a continuous and nonlinear relationship between median survival and extent of resection. This supports maximum safe resection for glioblastoma multiforme and challenges the commonly held dogma for avoiding subtotal resections for glioblastoma (Marko et al: J Clin Oncol 32:774–782, 2014).
Surgical tools. A variety of tools are available to help the neurosurgeon achieve these surgical goals, including stereotactic and image-based guidance systems and electrophysiologic brain mapping.
Stereotactic frames provide a rigid, three-dimensional (3D) coordinate system for accurate targeting of brain lesions identified on CT or MRI scans and are particularly well suited for obtaining tissue for biopsy from tumors located in sites where aggressive tissue removal would produce unacceptable neurologic deficits.
Image-based guidance system. “Frameless” or “image-guided” stereotactic systems use computer technology to coregister preoperative imaging studies with intraoperative head position, thereby establishing stereotactic accuracy without the need for a frame. These systems are useful for stereotactic biopsy or achieving maximal resections of predefined tumor volumes and minimizing surgical morbidity. Intraoperative MRI accomplishes similar goals but is limited by a requirement for specialized operating suites.
Intraoperative brain mapping, also termed “cortical mapping,” uses electrical stimulation of the cortical surface to define the primary motor, sensory, or speech cortex. By identifying the exact location of these areas before tumor resection, the surgeon can avoid these structures, thereby preserving neurologic function. These tools enable the neurosurgeon to perform more complete removal of tumors with less morbidity. Intraoperative MRI is being used in a number of centers to facilitate the complete removal of a tumor. Images are obtained before the operation is completed to ensure removal of all visible tumor.
Pathology-based surgical approach for primary brain tumors. The surgical approach to an intracranial lesion is strongly influenced by the suspected or previously confirmed pathology. Guidelines for the management of the most common tumors are discussed.
• Meningiomas and other extra-axial tumors-Benign extra-axial tumors, such as meningiomas, usually have a well-defined plane separating them from the surrounding brain parenchyma. In general, total extirpation can be achieved by open craniotomy, particularly when the tumor is located over the convexity. Firm attachment of the tumor to the dura, cranial nerves, vascular structures, or skull base may make this impossible. Subtotal resections that preserve neural or vascular structures while reducing mass effect are often favored for extensive skull base tumors.
The surgical management of other benign extra-axial tumors, such as acoustic neuroma, pineocytoma, choroid plexus papilloma, and pituitary adenoma, closely parallels that of meningiomas. Gross total resection is generally curative and should be attempted whenever it is safe.
• Low-grade gliomas-Gross total resection of all enhancing and non-enhancing disease, whenever possible, is the goal of surgery for low-grade gliomas and mixed neuronal-glial tumors (eg, astrocytoma, oligodendroglioma, pilocytic astrocytoma, and ganglioglioma). Long-term survival is better in patients who have undergone gross total resection than in those who have had subtotal resection (5-year survival rates > 80% for gross total resection vs approximately 50% for subtotal resection).
If radiographically proven gross total resection is attained, postoperative irradiation or chemotherapy can often be withheld until there is evidence of tumor progression (see section on “Radiation therapy”). If a postoperative scan reveals a small but surgically accessible residual lesion, immediate reoperation should be considered, particularly in children or in those with pilocytic astrocytomas (WHO grade I).
When low-grade tumors are found in patients with medically refractory chronic epilepsy, surgical management should be oriented toward curing the epilepsy as well as achieving total tumor removal.
• Ependymomas-Gross total resection is the goal of surgery whenever possible for ependymomas. Because ependymomas arise in the ventricular system, they can disseminate in the CSF. Therefore, all patients should be assessed for subarachnoid metastases with complete cranial and spinal MRI performed with gadolinium.
• High-grade gliomas-More extensive resections of enhancing disease are associated with improved quality of life and neurologic function in patients with high-grade gliomas (glioblastoma, anaplastic astrocytoma, and anaplastic oligodendroglioma) by reducing mass effect, edema, and corticosteroid dependence. Resection of more than 98% of the enhancing tumor volume prolongs survival relative to subtotal or partial resections. For this reason, most neurosurgeons attempt to achieve maximal resection while minimizing the risk to critical areas of the brain.
• Recurrent or progressive tumors-When a brain tumor recurs or enlarges, re-operation may often be necessary to reduce mass effect. Although rarely curative, these procedures can improve quality of life and modestly extend survival. In general, re-operation is not considered in patients with a Karnofsky performance status (KPS) score of 60 or less or in patients who are not candidates for additional therapy following surgery.
• Pseudoprogression-describes an increase in contrast enhancement independent of tumor growth in patients who have recently received radiation therapy. It occurs most frequently (58%) within the first 3 months following radiation therapy and is more commonly seen after concurrent chemoradiation therapy. It is estimated that up to 50% of patients with an increase in enhancement have true tumor progression. However, in practice, the patient’s current treatment regimen of temozolomide is usually continued for at least 3 months to avoid discontinuing an effective therapy. Pseudoprogression may represent a more robust response to therapy and may correlate with MGMT promoter methylation and a better therapeutic outcome. A recurrent tumor cannot be distinguished from radiation necrosis on routine MRI. Both disorders may cause severe mass effect and edema, and resection is the optimal treatment for both if the patient is symptomatic. Occasionally, PET or MRS can distinguish tumor from treatment effect, but these imaging modalities are unreliable.
Initial resection or reoperation followed by intracavitary or intraparenchymal administration of chemotherapy, immunotherapy, or liquid I-125 radiotherapy (GliaSite) is being explored but is still investigational. Carmustine-impregnated wafers (Gliadel) are the only form of intracavitary chemotherapy currently approved by the FDA for glioblastoma.
Radiation therapy
Radiation therapy plays a central role in the treatment of brain tumors in adults. It is the most effective nonsurgical therapy for patients with malignant gliomas and also has an important role in the treatment of patients with low-grade gliomas and metastatic brain tumors.
Whole-brain vs partial-brain irradiation. Whole-brain irradiation is reserved for multifocal lesions, lesions with significant subependymal or leptomeningeal involvement, and metastatic brain tumors. For the majority of patients with unifocal disease, limited-field treatment results in less morbidity and appears to produce equal, albeit poor, overall survival.
Intensity-modulated radiotherapy is an advanced technique to deliver high-precision radiotherapy to a tumor. Treatment is planned using 3D CT images to design a dose that will conform to the 3D shape of the tumor. Using multiple beams, a uniform dose of radiotherapy is delivered to the whole tumor while sparing normal tissues.
Radiation therapy for low-grade gliomas. Retrospective studies suggest a limited radiation dose response in low-grade gliomas. However, selection bias may play a role in these studies.
Several randomized studies addressed the question of optimal timing and dose of radiotherapy in patients with low-grade gliomas. An American intergroup randomized trial compared 50.4 Gy vs 64.8 Gy of radiation in patients with low-grade glioma. A European Organisation for Research and Treatment of Cancer (EORTC) trial compared 45 Gy vs 59.4 Gy of radiation in patients with low-grade astrocytoma. Both studies confirmed the superiority or equivalent efficacy of the lower radiation dose with less toxicity.
A second EORTC trial tested immediate vs delayed radiotherapy in individuals with low-grade glioma. Although immediate radiotherapy significantly improved 5-year progression-free survival, overall survival was identical in the two treatment arms. Furthermore, quality of life was better in patients whose radiotherapy was deferred until clinical or radiographic disease progression was evident.
Recommended treatment approach for low-grade astrocytomas. The role of postoperative radiotherapy in the management of incompletely resected low-grade astrocytomas has not been firmly established. However, on the basis of the available data, the following principles appear reasonable:
• Complete surgical resection of both enhancing and non-enhancing disease of hemispheric astrocytomas should be attempted.
• If complete surgical resection has been attained, radiation therapy can be withheld until MRI or CT studies clearly indicate a recurrence that cannot be approached surgically.
• When complete surgical resection is not performed, postoperative irradiation may be recommended, depending on the patients’ clinical condition.
• Radiation therapy should be delivered, using a megavoltage machine, in 1.7- to 2-Gy daily fractions, to a total dose of about 50 Gy. The treatment fields should include the primary tumor volume only, as defined by MRI, and should not encompass the whole brain.
• In low-grade astrocytomas, radiation therapy can be expected to produce a 5-year survival rate of 50% and a 10-year survival rate of 20%. Patients with low-grade oligodendrogliomas survive even longer.
• Cognitive impairment may develop in long-term survivors of low-grade gliomas. This may be due to the disease itself, surgical resection, antiepileptic drug use, and radiotherapy if used.
Radiation therapy for high-grade gliomas. An analysis of three studies of high-grade gliomas performed by the Brain Tumor Study Group showed that postoperative radiotherapy doses greater than 50 Gy were significantly better in improving survival than no postoperative treatment and that a dose of 60 Gy resulted in significantly prolonged survival compared with 50 Gy. Doses greater than 60 Gy used in the American Intergroup protocol resulted in competing morbidity.
On the basis of these data, involved-field radiotherapy to 60 Gy in 30 to 33 fractions is standard treatment for high-grade histologies; this amount corresponds to a dose just above the threshold for radionecrosis. About half of patients with anaplastic astrocytomas exhibit radiographic evidence of response following 60 Gy of radiation, compared with 25% of patients with glioblastoma. Complete radiographic response is rare in either case. Elderly patients with glioblastoma have a particularly poor prognosis, with a median survival of 4 to 6 months in most series. Some of these patients may elect not to undergo treatment. A randomized controlled study demonstrated that 50 Gy yields a significantly longer survival time than supportive care alone (median of 29.1 weeks vs 16.9 weeks; P = .002) without compromising quality of life. Hypofractionated radiotherapy was addressed in the elderly population in two other randomized studies, NOA-08 and the Nordic trial, further described in the section on chemotherapy for malignant gliomas. An abbreviated course of radiotherapy should be considered in patients older than 70 years.
Alternatives to conventional radiotherapy
The results of standard radiation treatment in patients with malignant gliomas are poor. Patients with glioblastoma have a median survival time of 15 months to 18 months, whereas patients with anaplastic astrocytomas survive a median of 3 to 5 years. To improve upon these poor results, a number of approaches have been tried, including hyperfractionated radiotherapy (HFRT), focal dose escalation with interstitial brachytherapy, and radiosurgery, but none has demonstrated improved survival in randomized controlled trials. Brachytherapy and HFRT have been abandoned. Radiosurgery is still considered in the recurrent setting; however, the evidence for this practice has not yet reached the strength of other treatment recommendations.
Radionecrosis. Both brachytherapy and stereotactic radiosurgery can induce focal radionecrosis. Concurrent chemotherapy further increases this risk. This complication produces symptoms of mass effect in about 50% of patients with malignant glioma, sometimes requiring resection to remove the necrotic debris. Occasionally, treatment with corticosteroids can control the edema around the radionecrotic area, but often the patient becomes corticosteroid-dependent, with all of the attendant complications of long-term corticosteroid use. Radionecrosis can be a significant limitation of the focal radiotherapy techniques.
Recommended approach for extra-axial tumors. Surgery alone is curative in the vast majority of patients with benign tumors. However, in certain subsets of patients, postoperative radiotherapy may control further growth of these lesions.
• Pituitary adenomas-For hormonally inactive pituitary adenomas that persist or recur after surgery, a radiation dose of 45 Gy to 50 Gy is delivered in 25 to 28 fractions to the radiographic boundaries of the tumor. For patients with Cushing disease and acromegaly, higher doses are required for biochemical remission. Coronal-enhanced MRI is critical for treatment planning, because CT often does not allow the clinician to visualize the skull base and the entire extent of disease.
The most common indications for radiotherapy are invasion of the cavernous sinus or the suprasellar space and incomplete resection of macroadenomas (> 1.5 cm). Most pituitary lesions do not grow following radiotherapy, and hormonally active tumors usually demonstrate a hormonal response, with a reduction in hormone hypersecretion in 1 to 3 years. Following radiation therapy, 20% to 50% of patients develop panhypopituitarism, requiring hormone replacement therapy. Other significant complications (ie, damage to the visual apparatus) are rare today.
• Meningiomas-are readily curable with complete surgical resection. However, base of skull lesions and lesions involving a patent venous sinus often cannot be resected completely. For some patients with these lesions, a course of postoperative radiotherapy is indicated. In general, a dose of 54 Gy is delivered in 30 fractions to the radiographic tumor region using 3D treatment planning. Malignant meningiomas always require postoperative radiotherapy, even after gross total resection. Radiosurgery may also be useful in treating meningiomas, and doses of 13 Gy to 18 Gy are associated with a high rate of control 10 years following therapy.
• Acoustic neuroma-has classically been considered a surgical disease. Following total resection, recurrence rates are less than 5%. When only subtotal resection is possible, disease recurs in at least 60% of patients.
Radiosurgery has been used as an alternative to surgery for acoustic neuroma. Tumor control rates of greater than 80% at 20 years have been reported. For patients with useful hearing before radiosurgery, that function is preserved in less than 50%. After radiosurgery, 10% of patients experience facial weakness, and 25% have trigeminal neuropathy. The risk of cranial neuropathies is related to the size of the lesion treated.
Chemotherapy
Malignant glioma. Chemotherapy has a limited but measurable benefit in the treatment of patients with malignant gliomas, and temozolomide and PCV (procarbazine, CCNU, and vincristine) are the most active regimens.
In a large phase III trial, patients with newly diagnosed glioblastoma were randomized to receive radiotherapy alone or radiotherapy with concurrently administered temozolomide followed by adjuvant temozolomide. A total of 573 patients were studied, and median survival was significantly prolonged, from 12.1 months to 14.6 months with the addition of temozolomide to radiotherapy. The 2-year survival rate was only 10% in those treated with radiotherapy alone compared with 27% in those who received radiotherapy plus temozolomide. The combined-modality regimen was well tolerated and was associated with minimal additional toxicity. The benefit of adding temozolomide to radiotherapy in newly diagnosed glioblastoma persists throughout 5 years of follow-up. This regimen is the standard for all patients with newly diagnosed glioblastoma and combines the potential radiosensitizing effect of concurrent temozolomide with the benefit of adjuvant chemotherapy.
This study demonstrates clear benefit in patients with glioblastoma, and many investigators have extrapolated this regimen for use in patients with gliomas of all grades. Current practice varies from treating anaplastic astrocytoma with radiation therapy alone, to sequential radiotherapy and chemotherapy, to concurrent chemoradiation therapy. Two ongoing phase III trials are addressing this issue.
In a nonrandomized phase II trial, 70 patients aged 70 years and older with glioblastoma and a KPS score of less than 70 were given temozolomide alone (150 to 200 mg/m2 daily for 5 days every 4 weeks) until progression. Grade 3 to 4 neutropenia and thrombocytopenia occurred in 13% and 14% of patients, respectively. Median progression-free survival time was 16 weeks and median overall survival time was 25 weeks, significantly greater than with supportive care alone, compared with historical controls. Eighteen patients (26%) increased their KPS score to 70 or greater.
The Radiation Therapy Oncology Group (RTOG) 0525 randomized 833 patients with glioblastoma to standard temozolomide (150 to 200 mg/m2 for 5 days) or dose-dense temozolomide (75 to 100 mg/m2 for 21 days) every 4 weeks for 6 to 12 cycles and found no difference in median overall survival, median progression-free survival, or methylation status. MGMT methylation was associated with improved overall survival (21.2 months vs 14 months; P < .0001) and progression-free survival (8.7 months vs 5.7 months; P < .0001), suggesting that standard-dose temozolomide should continue to be used outside a clinical trial for newly diagnosed glioblastoma. Those who received dose-dense temozolomide had increased grade (≥ 3) toxicity, primarily in those with lymphopenia and fatigue (19% vs 27%; P = .008).
The NOA-08 randomized phase III trial demonstrated both the noninferiority of temozolomide compared with radiation therapy in the treatment of elderly patients with high-grade glioma and the potential predictive utility of MGMT promoter methylation. Patients older than 65 years with newly diagnosed anaplastic astrocytoma or glioblastoma and a KPS greater than 60 were randomized to radiation therapy or temozolomide. Median overall survival and event-free survival did not differ between the study arms. Patients with MGMT methylation had longer event-free survival when treated with temozolomide (8.4 months vs 4.6 months), and those without promoter methylation had superior results with radiation therapy compared with temozolomide (4.6 months vs 3.3 months). This effect was also seen for overall survival.
The Nordic trial randomized glioblastoma patients 60 years of age and older to temozolomide, standard radiotherapy (60 Gy in 30 fractions), or hypofractionated radiotherapy (34 Gy in 10 fractions). Overall, patients receiving chemotherapy alone fared better, however those in the cohort of patients 60 to 70 years of age had equivalent survival in all three groups, and those in the older cohort aged > 70 years fared better with either temozolomide or hypofractionated radiotherapy.
Molecularly targeted agents have been a major focus of glioblastoma treatment in recent years. Randomized phase II trials have generated attention for their ability to expedite clinical testing of new agents. Unfortunately, targeted therapy has not been shown to be effective as single-agent therapy.
In a randomized phase II trial, cilengitide, an integrin receptor inhibitor, was given to patients with recurrent glioblastoma. Those receiving a dose of 2,000 mg twice weekly had an overall survival time of 9.9 months. In another randomized phase II trial, patients with progressive glioblastoma were given either erlotinib (Tarceva) or cytotoxic chemotherapy with temozolomide or carmustine. Erlotinib compared unfavorably, with progression-free survival at 6 months of only 11% vs 24% in the control group. EGFRvIII mutation did not correlate with efficacy.
A phase III trial randomized patients to receive enzastaurin, a protein kinase C beta inhibitor, or lomustine for progressive glioblastoma. The 6-month progression-free survival rate was 11% for enzastaurin and 19% for lomustine.
Targeting angiogenesis has proved to be an effective approach to prolong progression-free survival at recurrence. Bevacizumab (Avastin), a monoclonal antibody targeting vascular endothelial growth factor, received accelerated FDA approval for recurrent glioblastoma on the basis of two studies. In one study, patients with recurrent glioblastoma were given either bevacizumab alone or with irinotecan. Six-month progression-free and overall survival outcomes were 43% and 9.2 months, respectively, in the bevacizumab-alone group and 50% and 8.7 months in the combination group.
Two studies, AVAglio and RTOG 0825, examined the role of bevacizumab added to initial therapy for newly diagnosed glioblastoma. AVAglio showed significantly improved progression-free survival, while RTOG 0825 showed a trend toward increased progression-free survival but significance was not met. Neither trial showed an improvement in overall survival. While AVAglio showed improved quality of life, RTOG 0825 did not. The BELOB study randomized patients with recurrent glioblastoma to bevacizumab alone, lomustine alone, or the combination. Only the combination therapy met the prespecified criteria for 9-month overall survival at 59%, compared with 38% for bevacizumab alone and 43% for lomustine alone. This is the first study to demonstrate benefit to adding a chemotherapeutic agent to bevacizumab.
The GLARIUS trial randomized patients with newly diagnosed unmethylated glioblastoma to bevacizumab and irinotecan vs temozolomide, both in addition to standard radiotherapy. The 6-month progression-free survival was significantly improved for patients in the bevacizumab and irinotecan arm at 71.1% vs 26.2% for patients randomized to temozolomide (P < .0001).
Although using bevacizumab for unselected newly diagnosed patients was not shown to be superior, trials using bevacizumab for recurrent glioblastoma have shown increased response rates on MRI, improved 6-month progression-free survival and an improvement of neurological function and a reduction of steroids. For this reason it continues to be used in the treatment of glioblastoma. Thus far the only the addition of lomustine has been shown to be superior to bevacizumab monotherapy in this setting.
Disease progression after treatment with vascular-targeted agents may occur at the primary tumor site or at a distant site in the brain. It may have an atypical imaging pattern with diffuse infiltration that is nonenhancing and seen only on MRI FLAIR sequences or more recently described on diffusion-weighted sequences. There has been no increase in intratumoral hemorrhage with the antiangiogenic agents, which makes them safe for use in patients with malignant gliomas. Current studies are using multitargeted approaches to enhance efficacy.
Sidebar:Many practitioners fear tumor rebound upon cessation of bevacizumab. Anderson and colleagues reported on 82 patients treated with bevacizumab for more than 6 months. When 18 patients who stopped the drug for reasons other than progression were compared with those remaining on therapy, progression-free survival outcomes did not differ, and salvage was greater in those who had discontinued the treatment prior to tumor progression (progression-free survival at 26 weeks was 47% vs 5% (95% CI: 1%–21%), with a median progression-free survival time of 23 weeks vs 9 weeks (P = .0007)( Anderson et al: Neuro Oncol 16:823–828, 2014).
Astrocytoma. Until recently, many believed that chemotherapy had no role in the initial treatment of low-grade astrocytomas, but this practice is changing for selected high-risk astrocytomas.
Sidebar:RTOG 9802 randomized patients with ‘high-risk’ grade II glioma (defined as age > 40 years and/or less than completely resected) to either radiation therapy alone or radiation followed by chemotherapy. Updated results, with a median follow-up period of 12 years, showed a significant benefit for the addition of chemotherapy, with median overall survival of 13.3 years vs 7.8 years (P = .002) (Buckner et al: J Clin Oncol 32[suppl]; abstract 2000, 2014).
Oligodendroglioma. Oligodendroglioma is recognized as a particularly chemosensitive primary brain tumor. This finding was first observed with anaplastic oligodendroglioma but has recently been seen with the more common low-grade oligodendroglioma. Chemosensitivity of anaplastic and low-grade tumors is associated with loss of chromosomes 1p and 19q.
Several alkylating agents are active, but the best-studied regimen is PCV, which produces response rates of 75% and 90% in grade III and low-grade (grade II) oligodendrogliomas, respectively. PCV is an active regimen but has largely been supplanted by temozolomide because of the significant myelosuppression, neuropathy, and asthenia associated with PCV. Consequently, chemotherapy is an important therapeutic modality and may be used as initial treatment in patients with low-grade tumors who require therapeutic intervention. This approach defers or eliminates the late cognitive toxicity associated with cranial irradiation in patients with low-grade tumors who can have relatively prolonged survival.
RTOG 9402 and the EORTC trial 26951 demonstrated the significant impact of chemotherapy for newly diagnosed anaplastic oligodendrogliomas and anaplastic oligoastrocytomas (AOTs). RTOG 9402 randomized patients to chemotherapy with PCV followed by radiation therapy or to radiation therapy alone. Patients with 1p/19q co-deleted tumors who were treated with both chemotherapy and radiation therapy had a median survival time of 14.7 years, compared with a median survival time of 7.3 years for patients treated only with radiation therapy. EORTC 26951 randomized patients to radiation therapy alone or radiation followed by PCV. Survival for the entire cohort was greater in the chemotherapy-containing arm, at 42.3 months vs 30.6 months, and patients with 1p/19q co-deletion had a greater benefit of combined therapy with survival that was not reached after 140 months of follow-up vs 113 months. There was no survival difference for patients whose tumors were intact for 1p/19q.
Sidebar: Investigators of RTOG 9402 noted that patients with non-codeleted anaplastic oligodendroglial tumors who received chemotherapy in addition to radiation therapy had longer overall survival. When these patients were evaluated for IDH status, those with non-codeleted IDH-mutated tumors lived longer after receiving chemotherapy in addition to radiation (5.5 vs 3.3 years; P < .05) (Cairncross et al: J Clin Oncol 32:783–790, 2014).
Meningioma. Two independent reports describe the genomic alterations in meningioma. Focal NF2 gene inactivation was identified in 43% of tumors, and SMO or AKT1 mutations were identified in a subset of meningiomas lacking NF2 alterations. Non-NF2 meningiomas were usually benign, with chromosomal stability, and originating from medial aspects of the skull base. For the first time, these studies identify meningiomas with distinct genomic signatures and offer potential avenues for therapeutic targeting of these notoriously difficult to treat tumors.
Definitive Therapy: Brain Metastases
Surgery
Surgical approach for metastatic tumors. Most patients with brain metastases have a life expectancy of less than 6 months, but the majority who undergo resection of a metastatic lesion followed by irradiation will die of systemic rather than intracranial disease.
Excision of metastatic brain tumors is rarely curative, however, because microscopic cells may be left behind. Nevertheless, the reduced tumor burden becomes more amenable to adjuvant irradiation and/or chemotherapy.
Criteria. The decision whether to recommend surgery for metastatic brain tumors should be based on the following factors:
Extracranial oncologic status A comprehensive workup of the patient’s extracranial oncologic status is necessary. Extensive critical organ metastases preclude surgery in favor of palliative irradiation as the sole therapy. Brain surgery should not be performed in patients with limited expected survival (3 to 6 weeks) based on extracranial disease.
Number of metastases In general, only patients harboring a single metastasis are considered for resection. Occasionally, a large tumor will be removed in the presence of multiple smaller nodules if the edema and mass effect of this lesion are causing a substantial neurologic deficit that could be improved by tumor removal.
If brain metastasis is the presenting sign of systemic cancer and no clear primary source can be identified with routine staging, surgery may be required to establish a tissue diagnosis and plan further therapy.
In addition, surgical removal of a brain metastasis often reverses the neurologic deficits caused by compression of local structures by the tumor and reduces intracranial hypertension. After complete excision of a single brain metastasis, postoperative whole-brain radiotherapy improves control of neurologic disease but does not prolong survival.
Three studies have concluded that when multiple (up to three distinct locations) metastases are resected, either with or without radiotherapy, survival times are identical to those in patients with a surgically resected single metastasis and almost twice as long as those in patients treated by radiation therapy or radiosurgery alone. These studies suggest that a more aggressive surgical approach may be justified in patients with multiple brain metastases who have stable systemic disease.
Recurrence of solitary metastases. Up to 20% of single metastases may recur in long-term survivors. In these cases, a second operation may be warranted to remove the recurrent lesion and confirm the histologic diagnosis (ie, exclude radionecrosis).
The Graded Prognostic Assessment (GPA), a prognostic index for patients with brain metastases that traditionally included age, KPS, the presence or absence of extracranial metastases (ECM), and the number of brain metastases (NBM) was updated to reflect the heterogeneity of significant prognostic factors determined by primary tumor type. GPA scores range from 0 to 4, in order of worst to best prognosis. Factors include age, KPS, ECM, and NBM for both NSCLC and SCLC; KPS and NBM for melanoma and renal cell carcinoma; age, KPS, and tumor subtype for breast cancer; and KPS alone for gastrointestinal cancer (Figure 5).
FIGURE 5
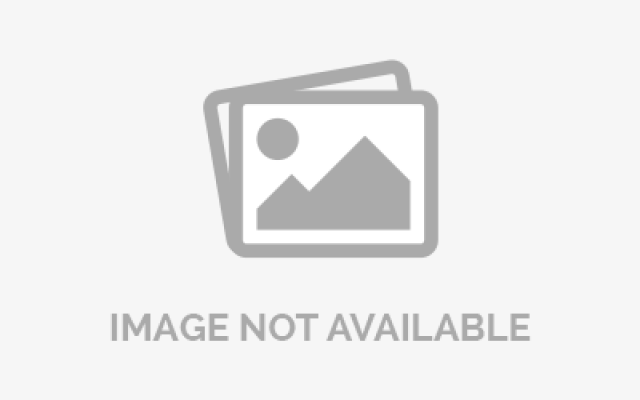
Graded Prognostic Assessment (GPA) worksheet to estimate survival from brain metastases (BM) by diagnosis
Radiotherapy
Radiation therapy for metastatic brain tumors. For symptomatic patients with brain metastases, median survival time is about 1 month if the patient remains untreated and 3 to 6 months if whole-brain radiation therapy is delivered, with no significant differences among various conventional radiotherapy fractionation schemes (20 Gy in 5 fractions, 30 Gy in 10 fractions, 40 Gy in 20 fractions). A more protracted schedule is used for patients who have limited or no evidence of systemic disease or for those who have undergone resection of a single brain metastasis, because these patients have the potential for long-term survival or even cure. The use of hypofractionated regimens is associated with an increased risk of neurologic toxicity.
The addition of the radiosensitizer motexafin gadolinium to whole-brain radiotherapy did not improve survival or time to neurologic disease progression in a randomized phase III trial. Subgroup analysis suggested a prolonged time to neurocognitive disease progression in patients with brain metastases from lung cancer, which was confirmed in a later study.
• Relief of neurologic symptoms-The major result of whole-brain radiation therapy is an improvement in neurologic symptoms, such as headache, motor loss, and impaired mentation. The overall response rate ranges from 70% to 90%. Unfortunately, symptomatic relief is not permanent, and symptoms recur with intracranial tumor progression.
• Multiple lesions-Patients with multiple lesions are generally treated with whole-brain radiation therapy alone. Re-treatment with a second course of whole-brain radiation therapy can provide further palliation for patients with progressive brain metastases (who have at least a 6-month or longer remission of symptoms after the initial course of cranial irradiation).
EORTC 22952 showed that adjuvant whole-brain radiation therapy decreases intracranial relapses and neurologic deaths but does not have an impact on survival or functional independence. Three hundred fifty-nine patients with one to three brain metastases underwent gross total resection or radiosurgery and were then randomly assigned to adjuvant whole-brain radiation therapy or observation. The median time to decline in performance status to more than 2 was 10 months after observation and 9.5 months after whole-brain radiation therapy, and overall survival was similar in both arms, at 10.7 months and 10.9 months, respectively.
• Concomitant corticosteroid therapy-Because the radiographic and clinical responses to whole-brain irradiation take several weeks, patients with significant mass effect should be treated with corticosteroids during whole-brain radiation therapy. Dexamethasone (16 mg/d) is started before therapy, and the dose may be tapered as tolerated during treatment. Occasionally, higher doses are necessary to ameliorate neurologic symptoms. However, most patients can be safely tapered off corticosteroids at the completion of whole-brain radiotherapy.
Radiosurgery for metastatic brain tumors
In patients with one to three brain metastases, aggressive local therapy (surgical resection or radiosurgery) produces superior survival and quality of life compared with whole-brain radiation therapy alone. Radiosurgery may be the optimal choice for elderly patients at greater risk for surgical morbidity. Radiosurgery has been used as sole therapy, as a boost to whole-brain radiation therapy, or for recurrent lesions in patients with brain metastases. Radiosurgery has the advantage of delivering effective focal treatment, usually in a single dose, without irradiating the normal brain. Radiosurgery of brain metastases less than 1 cm achieves 1- and 2-year local tumor control rates of 86% and 78%, respectively, significantly better than rates of 56% and 24%, respectively, for lesions greater than 1 cm. It is particularly useful for patients who have one to three lesions, each less than 4 cm in diameter. Patients with numerous lesions are not good candidates for radiosurgery because some of the ports may overlap, and more importantly, these patients likely harbor other microscopic lesions in the brain that are not being treated effectively with such focal therapy.
Brain metastases are particularly amenable to treatment with radiosurgery. Metastatic tumors do not infiltrate the brain and tend to have well-circumscribed borders; therefore, they can be targeted effectively with highly focused irradiation techniques that maintain a sharp delineation between the enhancing tumor seen on neuroimaging and normal brain. Furthermore, radiosurgery does not have the operative morbidity that may be associated with resection of a brain metastasis. Consequently, it can be used safely in many patients who are not surgical candidates, and it can even treat lesions in surgically unapproachable locations such as the brain stem.
Radiosurgery can achieve crude local tumor control rates of 73% to 98% over a median follow-up periodof 5 to 26 months. Radiosurgery was initially used as a boost after treatment with whole-brain radiotherapy. Three randomized trials have reported on the value of radiosurgery in addition to whole-brain radiotherapy for patients with multiple brain metastases. Although all three studies show a local tumor control advantage and an improvement in quality-of-life endpoints with the addition of a radiosurgical boost, none shows a statistical advantage in survival. For patients with multiple brain metastases, adding radiosurgery to whole-brain radiotherapy only offers an improved neurologic quality of life with no impact on survival.
A prospective, randomized RTOG trial compared whole-brain radiotherapy alone vs whole-brain radiotherapy plus radiosurgery in patients with one to three metastases. Although there was no statistical improvement in overall survival in the two arms of the trial, a subset analysis showed improved survival for those patients with a single lesion. Local tumor control, neurologic function, and corticosteroid doses were improved in patients with a single lesion treated with radiosurgery.
Radiosurgery is often considered an alternative to standard surgical resection, and accumulating evidence suggests they are largely equivalent for smaller lesions (< 2 cm), with some important nuances tailored to individual patient situations. Most retrospective studies suggest that the two techniques produce similar results; however, some reports indicate that surgery offers improved local tumor control, whereas others suggest that radiosurgery is superior.
Increasingly, radiosurgery is being used as the sole initial therapy for patients with one to three brain metastases. A prospective randomized trial compared radiosurgery with or without whole-brain radiotherapy in patients with one to four brain metastases. Results were similar to those of the phase III trial of surgical resection of a single brain metastasis with or without radiotherapy: improved local tumor control but no survival benefit. Therefore, whole-brain radiotherapy reduced CNS relapse but had no impact on survival.
Median survival from the time of radiosurgery is 6 to 15 months, and some patients can live for years without recurrence. Most patients exhibit clinical improvement and decreased corticosteroid requirement after radiosurgery, and only 11% to 25% of patients eventually die of neurologic causes.
Chemotherapy
Metastatic brain tumors. Chemotherapy usually has a limited role in the treatment of brain metastases and has not proved to be effective as adjuvant therapy after irradiation or surgery. However, it may have some efficacy in patients with recurrent brain metastases who are not eligible for further whole-brain radiation therapy or stereotactic radiosurgery. In addition, chemotherapy has proved active in patients with asymptomatic brain metastases (discovered on screening neuroimaging) who are scheduled to receive chemotherapy for their systemic disease. We have seen patients with brain metastases from a variety of primary tumors respond in this situation. A recent phase III trial of chemotherapy with early vs delayed whole-brain radiotherapy in NSCLC patients with brain metastases showed an identical intracranial response rate and survival. Thus, systemic chemotherapy had some efficacy against brain metastases.
A recently completed phase II trial of temozolomide (75 mg/m2/d) and concurrent whole-brain radiotherapy (40 Gy in 20 fractions) vs whole-brain radiotherapy alone demonstrated improved response rates and neurologic improvement in the combined-modality arm. In addition, there is growing recognition that systemic chemotherapy, including targeted agents, can be effective against brain metastases when the drugs are selected based on the primary tumor. Capecitabine (Xeloda), used primarily with lapatinib, is effective against brain metastases from HER2-positive breast cancer. High-dose methotrexate has activity against a number of primary tumors. Temozolomide has activity against recurrent brain metastases, particularly from NSCLC and melanoma.
Erlotinib has efficacy against brain metastases from lung cancer with the appropriate EGFR mutations.
Suggested Reading
On Primary Intracranial Tumors
Anderson MD, Hamza MA, Hess KR, et al: Implications of bevacizumab discontinuation in adults with recurrent glioblastoma. Neuro Oncol 16:823–828, 2014.
Brennan CW, Verhaak RG, McKenna A, et al: The somatic genomic landscape of glioblastoma. Cell 155:462–477, 2013.
Buckner JC, Pugh SL, Shaw EG, et al: Phase III study of radiation therapy (RT) with or without procarbazine, CCNU, and vincristine (PCV) in low-grade glioma: RTOG 9802 with Alliance, ECOG, and SWOG. J Clin Oncol 32(suppl):abstr 2000, 2014.
Cairncross JG, Wang M, Jenkins RB, et al: Benefit from procarbazine, lomustine, and vincristine in oligodendroglial tumors is associated with mutation of IDH. J Clin Oncol 32:783–790, 2014.
Cairncross G, Wang M, Shaw E, et al: Phase III trial of chemoradiotherapy for anaplastic oligodendroglioma: Long-term results of RTOG 9402. J Clin Oncol 31:337–343, 2012.
Gállego Pérez-Larraya J,Ducray F, Chinot O, et al: Temozolomide in elderly patients with newly diagnosed glioblastoma and poor performance status: an ANOCEF phase II trial. J Clin Oncol 29:3050–3055, 2011.
Gorovets D, Kannan K, Shen R, et al: IDH mutation and neuroglial developmental features define clinically distinct subclasses of lower grade diffuse astrocytic glioma. Clin Cancer Res 18:2490–2501, 2012.
Lai A, Kharbanda S, Pope WB, et al: Evidence for sequenced molecular evolution of IDH1 mutant glioblastoma from a distinct cell of origin. J Clin Oncol 29:4482–4490, 2011.
Malmström A, Grønberg BH, Marosi C, et al: Temozolomide versus standard 6-week radiotherapy versus hypofractionated radiotherapy in patients older than 60 years with glioblastoma: The Nordic randomised, phase 3 trial. Lancet Oncol 13:916–926, 2012.
Marko NF, Weil RJ, Schroeder JL, et al: Extent of resection of glioblastoma revisited: personalized survival modeling facilitates more accurate survival prediction and supports a maximum-safe-resection approach to surgery. J Clin Oncol 32:774–782, 2014.
Northcott PA, Korshunov A, Witt H, et al: Medulloblastoma comprises four distinct molecular variants. J Clin Oncol 29:1408–1414, 2011.
Remke M, Hielscher T, Northcott PA, et al: Adult medulloblastoma comprises three major molecular variants. J Clin Oncol 29:2717–2723, 2011.
van den Bent MJ, Brandes AA, Taphoorn MJ, et al: Adjuvant procarbazine, lomustine, and vincristine chemotherapy in newly diagnosed anaplastic oligodendroglioma: Long-term follow-up of EORTC brain tumor group study 26951. J Clin Oncol 31:344–350, 2012.
Wen PY, Macdonald DR, Reardon DA, et al: Updated response assessment criteria for high-grade gliomas: Response assessment in neuro-oncology working group. J Clin Oncol 28:1963–1972, 2010.
Wick W, Platten M, Meisner C, et al: Temozolomide chemotherapy alone versus radiotherapy alone for malignant astrocytoma in the elderly: The NOA-08 randomised, phase 3 trial. Lancet Oncol 13:707–715, 2012.
On Primary Extra-Axial Tumors
Brastianos PK, Horowitz PM, Santagata S, et al: Genomic sequencing of meningiomas identifies oncogenic SMO and AKT1 mutations. Nat Genet 45:285–289, 2013.
Clark VE, Erson-Omay EZ, Serin A, et al: Genomic analysis of non-NF2 meningiomas reveals mutations in TRAF7, KLF4, AKT1, and SMO. Science 339:1077–1080, 2013.
On Metastatic Brain Tumors
Chang EL, Wefel JS, Hess KR, et al: Neurocognition in patients with brain metastases treated with radiosurgery or radiosurgery plus whole-brain irradiation: A randomised controlled trial. Lancet Oncol 10:1037–1044, 2009.
Kocher M, Soffietti R, Abacioglu U, et al: Adjuvant whole-brain radiotherapy versus observation after radiosurgery or surgical resection of one to three cerebral metastases: Results of the EORTC 22952-26001 study. J Clin Oncol 29:134–141, 2011.
Lin NU, Dieras V, Paul D, et al: Multicenter phase II study of lapatinib in patients with brain metastases from HER2-positive breast cancer. Clin Cancer Res 15:1452–1459, 2009.
Sperduto PW, Kased N, Roberge D, et al: Summary Report on the Graded Prognostic Assessment: An accurate and facile diagnosis-specific tool to estimate survival for patients with brain metastases. J Clin Oncol 30:419–425, 2012.
Sun A, Bae K, Gore EM, et al: Phase III trial of prophylactic cranial irradiation compared with observation in patients with locally advanced non-small-cell lung cancer: Neurocognitive and quality-of-life analysis. J Clin Oncol 29:279–286, 2011.
Newsletter
Stay up to date on recent advances in the multidisciplinary approach to cancer.